Gut/Brain Communication in Health and Diseases
Our lab uses advanced technologies, genetically engineered models, ex-vivo cultures, electrophysiology, calcium imaging and human-derived cell lines to unmask pathways regulating bodyweight, glucose and somatosensory functions. Sensing of gut metabolites by the peripheral nerve system (PNS) is altered in states such as obesity, aging, or even neurodegenerative diseases (neuropathic pain, Parkinson etc.). We strive to translate our research to human to develop therapies. Summer 2022, our lab relocated from Chicago to Lausanne.
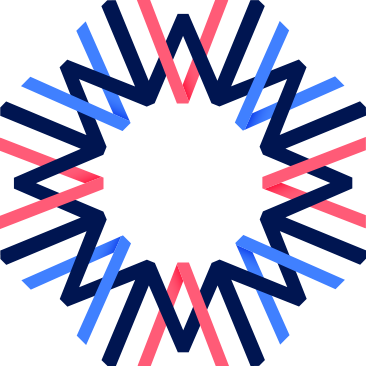
The specific research directions
of our laboratory include
Project 1
How prebiotics lead to better energy balance and cognitive function?
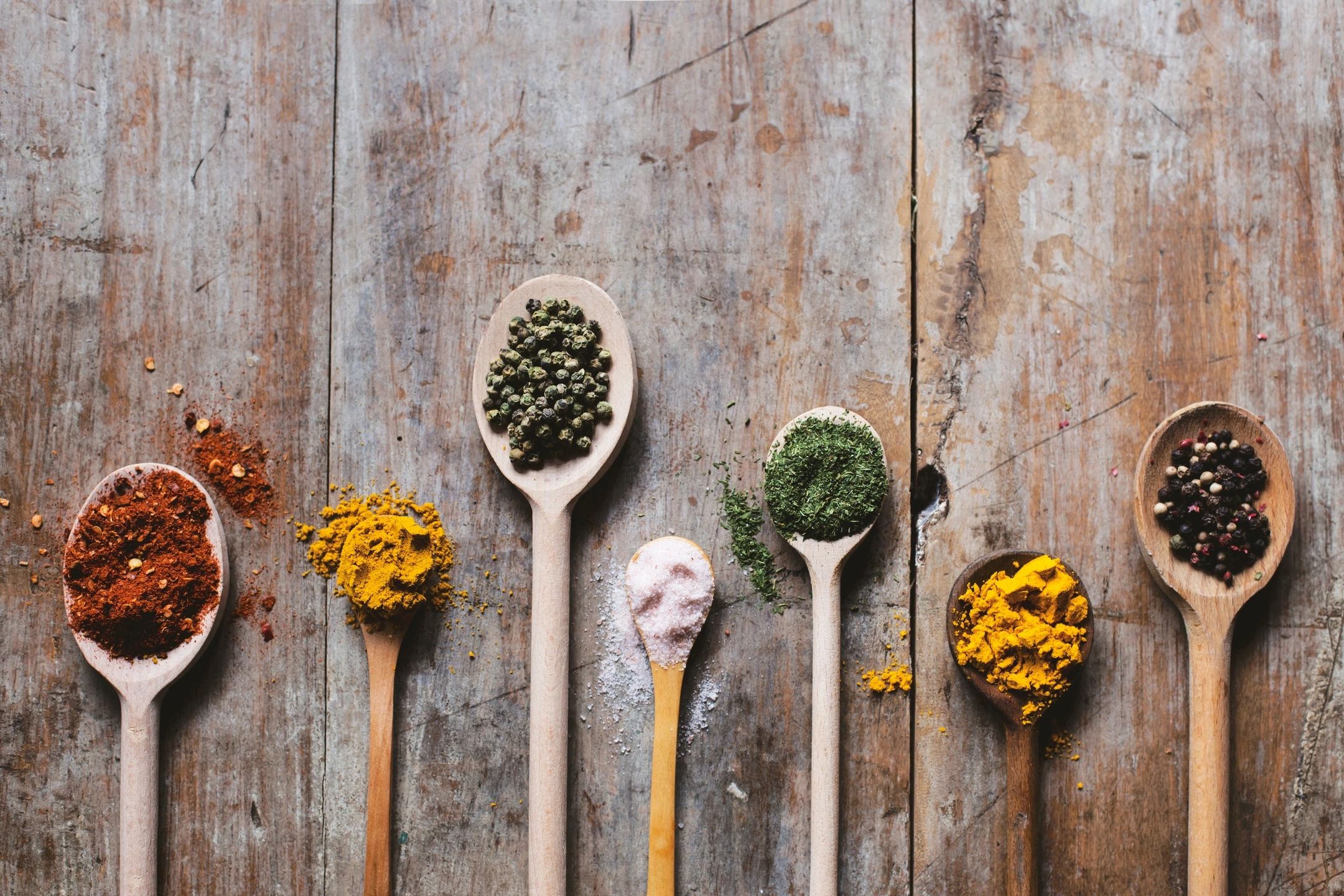
Prebiotics, a type of dietary fiber, play a crucial role in promoting better energy balance and cognitive function. Here’s how they achieve these benefits:
1. Improved Gut Health and Metabolic Function
Gut Microbiota Modulation: Prebiotics are non-digestible food ingredients that stimulate the growth and activity of beneficial gut bacteria. By promoting a healthy gut microbiota, prebiotics enhance nutrient absorption and energy extraction from food. Key beneficial bacteria like Bifidobacteria and Lactobacilli thrive on prebiotics, leading to improved gut health.
Short-Chain Fatty Acids (SCFAs) Production: Prebiotics are fermented by gut bacteria, producing SCFAs such as acetate, propionate, and butyrate. SCFAs serve as an energy source for colon cells, reduce inflammation, and regulate appetite by influencing hormone release (e.g., ghrelin and peptide YY). This can lead to better energy homeostasis and reduced fat accumulation.
2. Regulation of Appetite and Energy Intake
Hormonal Regulation: SCFAs influence the release of hormones involved in appetite regulation, such as glucagon-like peptide-1 (GLP-1) and peptide YY (PYY). These hormones increase satiety and reduce overall calorie intake, contributing to better energy balance.
Reduced Inflammation: Chronic inflammation is linked to obesity and metabolic disorders. Prebiotics help reduce systemic inflammation by promoting a healthy gut barrier and reducing the translocation of pro-inflammatory compounds. This, in turn, supports better metabolic function and energy balance.
3. Enhanced Cognitive Function
Gut-Brain Axis: The gut-brain axis is the bidirectional communication network between the gut and the brain. Prebiotics positively influence this axis by modulating gut microbiota composition and reducing gut inflammation, which can improve brain function and mood.
Neurotransmitter Production: Gut bacteria produce neurotransmitters such as serotonin and gamma-aminobutyric acid (GABA), which play a role in mood regulation and cognitive function. A healthy gut microbiota, supported by prebiotics, ensures the optimal production and balance of these neurotransmitters.
Brain-Derived Neurotrophic Factor (BDNF): SCFAs can cross the blood-brain barrier and promote the expression of BDNF, a protein crucial for neurogenesis, cognitive function, and mental health. Increased BDNF levels are associated with improved memory, learning, and mood.
Stress and Anxiety Reduction: Prebiotics can reduce stress and anxiety by influencing the hypothalamic-pituitary-adrenal (HPA) axis. A balanced HPA axis results in better stress management and improved cognitive function.
4. Indirect Benefits on Cognitive Function
Enhanced Sleep Quality: A healthy gut microbiota can improve sleep quality, which is essential for cognitive function. Better sleep supports memory consolidation, learning, and overall brain health.
Improved Immune Function: A robust immune system, supported by a healthy gut microbiota, reduces the risk of infections and illnesses that can negatively impact cognitive function. Prebiotics help maintain a balanced immune response.
Conclusion
Prebiotics contribute to better energy balance and cognitive function through their positive effects on gut health, hormonal regulation, and the gut-brain axis. By promoting a healthy gut microbiota, reducing inflammation, and supporting neurotransmitter production, prebiotics help optimize metabolic function and enhance cognitive performance. Integrating prebiotic-rich foods like garlic, onions, bananas, and whole grains into the diet can lead to these beneficial outcomes.
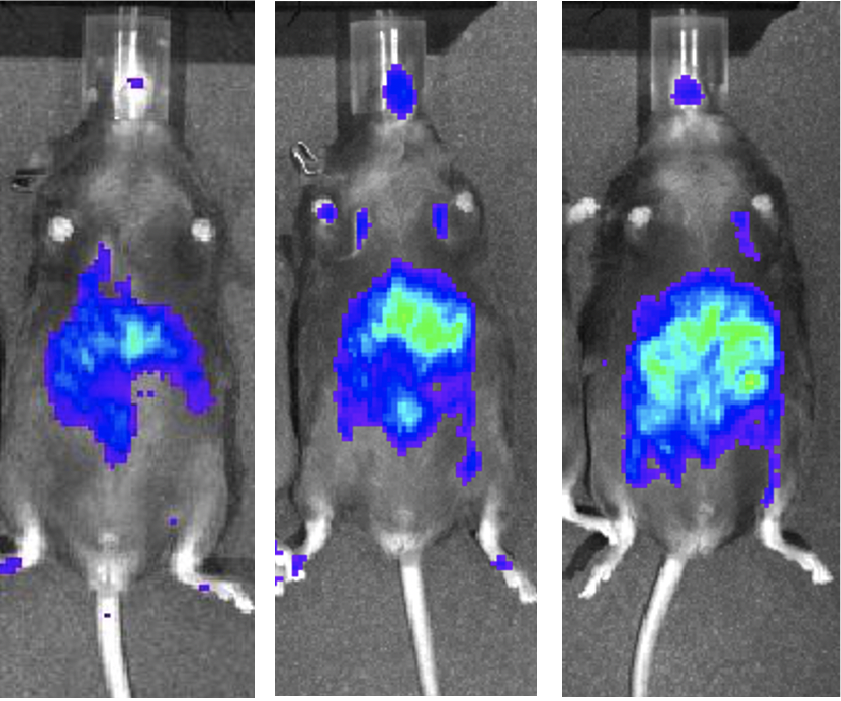
Project 2
Role of short chain fatty acid in diet-induced neuropathies.
How gut microbiome can impact the peripheral nerve system and associated diseases such as pain?
SCFAs and Gut-Brain Axis
- Gut-Brain Communication: SCFAs are critical mediators in the gut-brain axis, influencing brain function and behavior. They can cross the blood-brain barrier and interact with receptors in the nervous system.
- Neuroinflammation: SCFAs have anti-inflammatory properties. They can modulate the immune response by inhibiting histone deacetylases (HDACs), leading to reduced neuroinflammation, which is a key factor in the development of neuropathies.
Mechanisms of Action in Neuropathies
- Anti-inflammatory Effects: SCFAs reduce the production of pro-inflammatory cytokines and promote the production of anti-inflammatory cytokines. This helps in mitigating inflammation-induced nerve damage.
- Neuroprotective Effects: SCFAs promote neurogenesis and protect against neuronal damage. For example, butyrate has been shown to enhance brain-derived neurotrophic factor (BDNF) expression, which supports neuronal survival and growth.
- Modulation of Pain Perception: SCFAs influence pain perception by acting on the central and peripheral nervous systems. They can modulate the activity of pain receptors and reduce hypersensitivity, which is often associated with neuropathies.
Impact on Diet-Induced Neuropathies
- Diabetic Neuropathy: In diabetes, SCFAs help in maintaining glucose homeostasis and reducing inflammation. Butyrate, in particular, has shown protective effects against diabetic neuropathy by improving metabolic parameters and reducing oxidative stress.
- Obesity-Related Neuropathy: Obesity can lead to neuropathy through inflammatory pathways. SCFAs help in reducing obesity-induced inflammation and improving gut barrier function, thereby reducing the risk of neuropathy.
- Chemotherapy-Induced Neuropathy: Some studies suggest that SCFAs can alleviate chemotherapy-induced neuropathy by reducing neuroinflammation and oxidative stress.
Dietary Interventions
- High-Fiber Diets: Diets rich in fibers promote the production of SCFAs. Foods such as fruits, vegetables, whole grains, and legumes are beneficial.
- Probiotics and Prebiotics: These can enhance SCFA production by promoting a healthy gut microbiome. Probiotic supplements and prebiotic-rich foods (like garlic, onions, and bananas) can be effective.
Project 3
Gut-brain communication involving vagal and spinal sensing of short chain fatty acids/ portal sensing
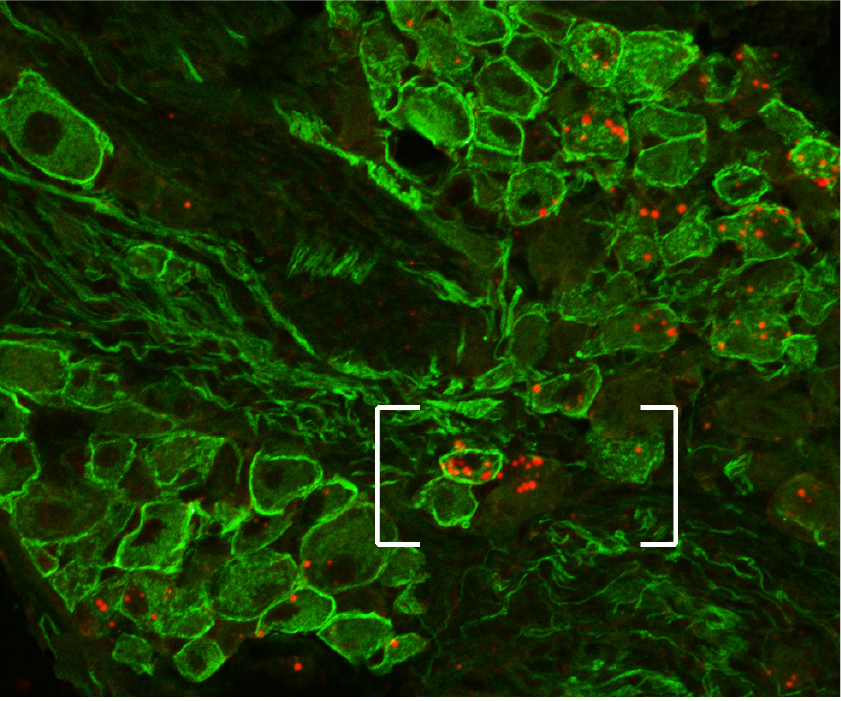
Gut-brain communication is a complex and intricate system involving multiple pathways that relay information from the gut to the brain and vice versa. Short chain fatty acids (SCFAs), which are produced by the fermentation of dietary fibers by gut microbiota, play a significant role in this communication network. Here’s a detailed overview of how SCFAs are involved in gut-brain communication, focusing on vagal and spinal sensing, as well as portal sensing mechanisms:
Vagal Sensing of SCFAs
The vagus nerve is a crucial component of the parasympathetic nervous system, connecting the brain to various organs, including the gut. It plays a pivotal role in sensing gut-derived signals and transmitting them to the brain.
- Vagal Afferent Neurons: SCFAs can influence vagal afferent neurons directly or indirectly. These neurons have receptors that can detect changes in the gut environment.
- SCFA Receptors: G-protein-coupled receptors (GPCRs) such as GPR41 (also known as FFAR3) and GPR43 (FFAR2) are activated by SCFAs. These receptors are expressed on enteroendocrine cells and vagal afferent neurons.
- Enteroendocrine Cells: SCFAs stimulate enteroendocrine cells to release gut hormones (such as GLP-1 and PYY), which can activate vagal afferent pathways. This hormonal signaling modulates appetite, satiety, and energy homeostasis.
- Neurotransmitter Release: Activation of vagal afferent neurons by SCFAs can lead to the release of neurotransmitters like acetylcholine, which communicates with the central nervous system to regulate physiological responses.
Spinal Sensing of SCFAs
The spinal cord also plays a role in transmitting gut-derived signals to the brain, particularly through the spinal afferent pathways.
- Spinal Afferent Neurons: These neurons can sense SCFAs and other metabolites in the gut, relaying information to the brain about the gut’s state.
- Inflammatory and Pain Responses: SCFAs can modulate inflammatory responses and pain perception through spinal afferent pathways. They help reduce neuroinflammation and pain, which are often associated with various neuropathies.
Portal Sensing of SCFAs
The portal vein carries blood from the gastrointestinal tract to the liver, and it plays a key role in sensing and metabolizing SCFAs.
- Hepatic Portal Vein: SCFAs absorbed from the gut enter the hepatic portal vein and reach the liver, where they can influence metabolic processes and liver function.
- Liver-Brain Axis: The liver communicates with the brain via various signaling molecules, including SCFAs. The liver’s metabolic state, influenced by SCFAs, can affect systemic inflammation and metabolic regulation.
- SCFA Metabolism: In the liver, SCFAs are metabolized to provide energy or act as signaling molecules. Acetate, for instance, can be converted to acetyl-CoA, entering the tricarboxylic acid cycle for energy production.
Integrated Gut-Brain Communication
- Neuroimmune Modulation: SCFAs influence the immune system by modulating the production of cytokines and other immune cells. This neuroimmune interaction can affect brain function and behavior.
- Microbiota-Gut-Brain Axis: The gut microbiota produce SCFAs, which interact with the nervous system through the mechanisms mentioned above. This axis plays a critical role in maintaining homeostasis and responding to dietary changes.
- Behavioral and Cognitive Effects: SCFAs can influence brain function directly (by crossing the blood-brain barrier) or indirectly (via vagal and spinal pathways), affecting mood, cognition, and behavior.
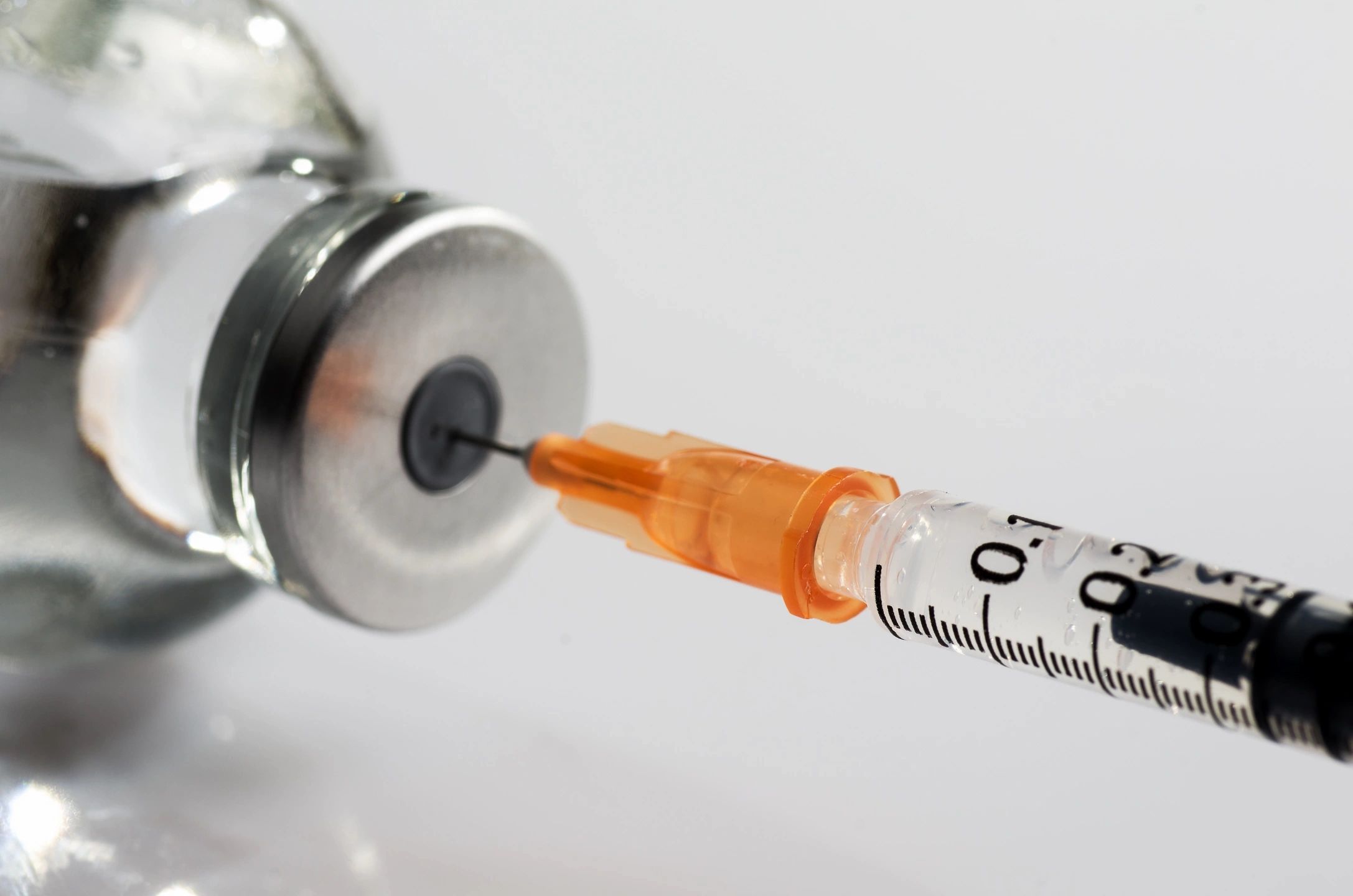
Project 4
How short chain fatty acids interact with immune cells to impact energy balance and glucose homeostasis?
SCFAs and Immune Cell Interaction
- Receptor Activation:
- GPCRs: SCFAs interact with G-protein-coupled receptors (GPCRs), specifically GPR41 (FFAR3) and GPR43 (FFAR2), which are expressed on immune cells such as macrophages, neutrophils, and dendritic cells.
- Histone Deacetylase (HDAC) Inhibition: SCFAs, especially butyrate and propionate, act as HDAC inhibitors, affecting gene expression and function in immune cells.
- Anti-inflammatory Effects:
- Cytokine Production: SCFAs reduce the production of pro-inflammatory cytokines (like TNF-α, IL-6, and IL-1β) and increase anti-inflammatory cytokines (such as IL-10) by immune cells, promoting a balanced immune response.
- Regulatory T Cells (Tregs): SCFAs enhance the differentiation and function of Tregs, which play a critical role in maintaining immune tolerance and suppressing excessive inflammatory responses.
Impact on Energy Balance
- Adipose Tissue Function:
- Adipogenesis: SCFAs, particularly butyrate, promote adipogenesis, which is the formation of new fat cells. This helps in storing excess energy and regulating lipid metabolism.
- Thermogenesis: SCFAs stimulate the expression of uncoupling protein 1 (UCP1) in brown adipose tissue (BAT), enhancing thermogenesis and energy expenditure.
- Appetite Regulation:
- Gut Hormones: SCFAs stimulate the release of gut hormones like peptide YY (PYY) and glucagon-like peptide-1 (GLP-1), which promote satiety and reduce appetite.
- Vagal Pathways: SCFAs activate vagal afferent neurons, signaling the brain to modulate appetite and food intake.
Impact on Glucose Homeostasis
- Insulin Sensitivity:
- Anti-inflammatory Action: By reducing inflammation, SCFAs improve insulin signaling pathways in tissues, enhancing insulin sensitivity.
- Adipocyte Function: SCFAs regulate adipocyte differentiation and function, improving insulin sensitivity and glucose uptake by adipose tissues.
- Hepatic Gluconeogenesis:
- Gene Expression: SCFAs, especially butyrate, inhibit hepatic gluconeogenesis by downregulating the expression of genes involved in glucose production in the liver.
- Energy Substrates: SCFAs provide substrates for energy production in the liver, reducing the need for glucose production from non-carbohydrate sources.
- Pancreatic Function:
- Insulin Secretion: SCFAs enhance the secretion of insulin from pancreatic β-cells in response to glucose, improving glucose homeostasis.
- Islet Inflammation: By reducing inflammation in pancreatic islets, SCFAs protect β-cells from dysfunction and apoptosis.
Mechanisms of Action
- Epigenetic Modulation:
- HDAC Inhibition: SCFAs inhibit HDACs, leading to an open chromatin structure and increased transcription of genes involved in metabolic processes and immune regulation.
- Histone Acetylation: Increased histone acetylation in immune cells alters the expression of genes that regulate inflammation and metabolism.
- Metabolic Pathways:
- AMP-Activated Protein Kinase (AMPK): SCFAs activate AMPK, a key energy sensor that enhances glucose uptake, fatty acid oxidation, and mitochondrial biogenesis.
- mTOR Pathway: SCFAs can inhibit the mTOR pathway, which is involved in cell growth and metabolism, promoting autophagy and reducing insulin resistance.
Project 5
SCFA and mother/embryo interaction : impact on energy and glucose homeostasis
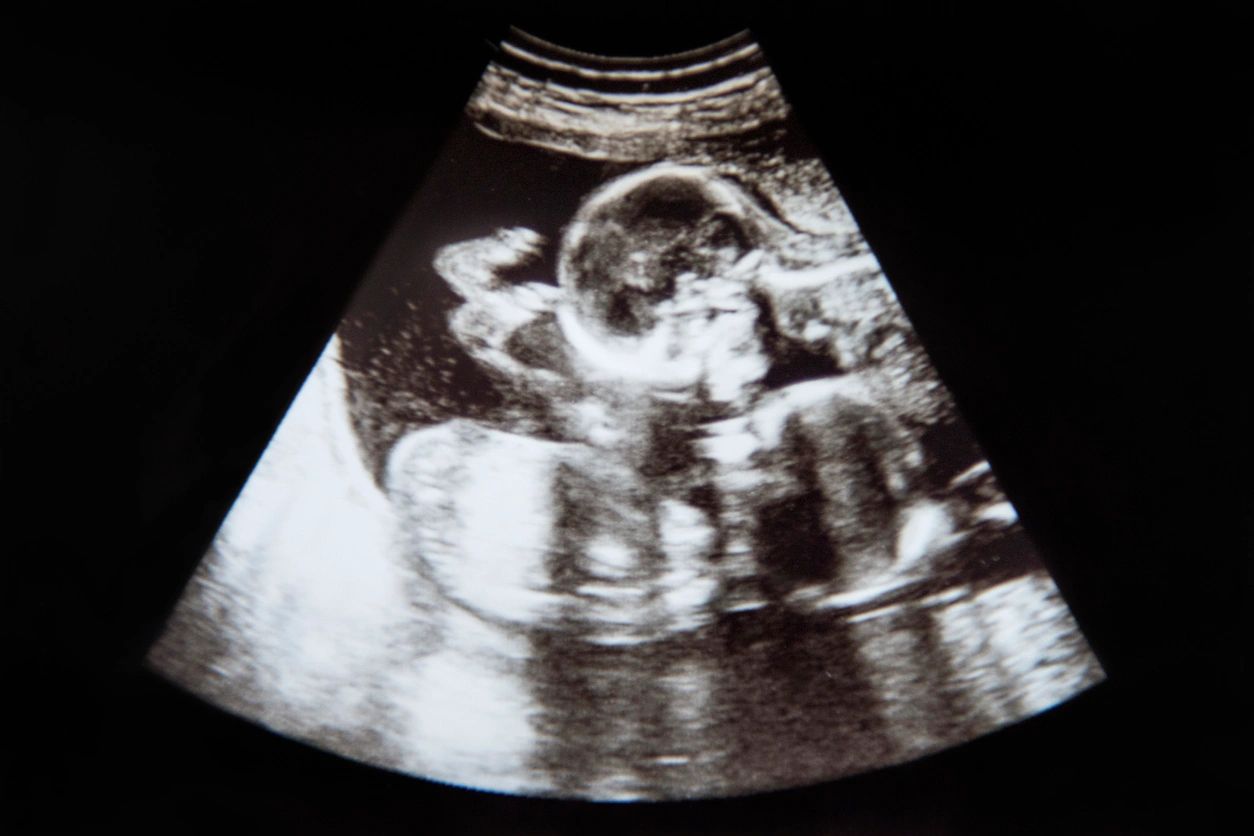
Short chain fatty acids (SCFAs) play a crucial role in maternal and fetal health, particularly in the context of energy balance and glucose homeostasis. The interaction between SCFAs and the mother/embryo axis influences several physiological processes that are critical during pregnancy. Here’s an in-depth look at how SCFAs impact this interaction:
Maternal-Fetal Interaction and SCFAs
- Gut Microbiota and SCFAs:
- Maternal Microbiota: The maternal gut microbiota produces SCFAs through the fermentation of dietary fibers. These SCFAs enter the maternal circulation and can cross the placenta to reach the fetus.
- Fetal Exposure: SCFAs are present in the amniotic fluid and can be ingested by the fetus, influencing fetal development and metabolism.
- Placental Function:
- Nutrient Transport: SCFAs influence the function of the placenta, including the transport of nutrients and metabolites to the fetus. This ensures that the fetus receives adequate energy and nutrients for growth.
- Placental Inflammation: SCFAs have anti-inflammatory properties that can reduce placental inflammation, which is crucial for maintaining a healthy pregnancy environment.
Impact on Energy Balance
- Maternal Energy Metabolism:
- Increased Energy Demand: Pregnancy increases the energy demands of the mother. SCFAs help meet these demands by modulating energy metabolism, enhancing mitochondrial function, and promoting lipid oxidation.
- Adipose Tissue: SCFAs promote the storage of energy in adipose tissue, ensuring that the mother has adequate energy reserves to support fetal growth.
- Fetal Energy Metabolism:
- Growth and Development: SCFAs provide an energy source for the developing fetus. Butyrate, in particular, is utilized by fetal tissues for energy production.
- Fat Deposition: SCFAs influence the deposition of fat in fetal tissues, which is important for energy storage and thermoregulation after birth.
Impact on Glucose Homeostasis
- Maternal Glucose Homeostasis:
- Insulin Sensitivity: SCFAs improve maternal insulin sensitivity by reducing inflammation and enhancing insulin signaling pathways. This helps in maintaining normal blood glucose levels during pregnancy.
- Gestational Diabetes: Adequate production of SCFAs through a high-fiber diet may reduce the risk of gestational diabetes by modulating glucose metabolism and improving insulin sensitivity.
- Fetal Glucose Homeostasis:
- Insulin Secretion: SCFAs can influence the development of the fetal pancreas and the secretion of insulin. Proper insulin function is crucial for fetal glucose regulation and growth.
- Glucose Transport: SCFAs enhance the expression of glucose transporters in the placenta, ensuring efficient transfer of glucose from the mother to the fetus.
Mechanisms of Action
- Receptor Activation:
- GPCRs: SCFAs activate GPR41 and GPR43 on maternal and fetal tissues, influencing metabolic processes and immune responses that are critical for energy and glucose homeostasis.
- HDAC Inhibition: By inhibiting histone deacetylases, SCFAs modulate gene expression in both maternal and fetal cells, promoting anti-inflammatory and metabolic pathways.
- Hormonal Regulation:
- Gut Hormones: SCFAs stimulate the release of gut hormones like GLP-1 and PYY, which regulate maternal appetite, insulin secretion, and glucose homeostasis.
- Placental Hormones: SCFAs may influence the production of placental hormones that regulate fetal growth and energy metabolism.
Implications for Pregnancy and Offspring Health
- Maternal Diet:
- High-Fiber Diet: Consuming a diet rich in dietary fibers enhances SCFA production, benefiting both maternal and fetal health by improving energy balance and glucose homeostasis.
- Probiotics and Prebiotics: Supplementing with probiotics and prebiotics can further enhance SCFA production and promote a healthy gut microbiota during pregnancy.
- Long-Term Health:
- Metabolic Programming: Exposure to SCFAs in utero can have long-term effects on the offspring’s metabolism, potentially reducing the risk of metabolic disorders like obesity and diabetes later in life.
- Immune Development: SCFAs play a role in the development of the fetal immune system, which can influence the immune health of the offspring.
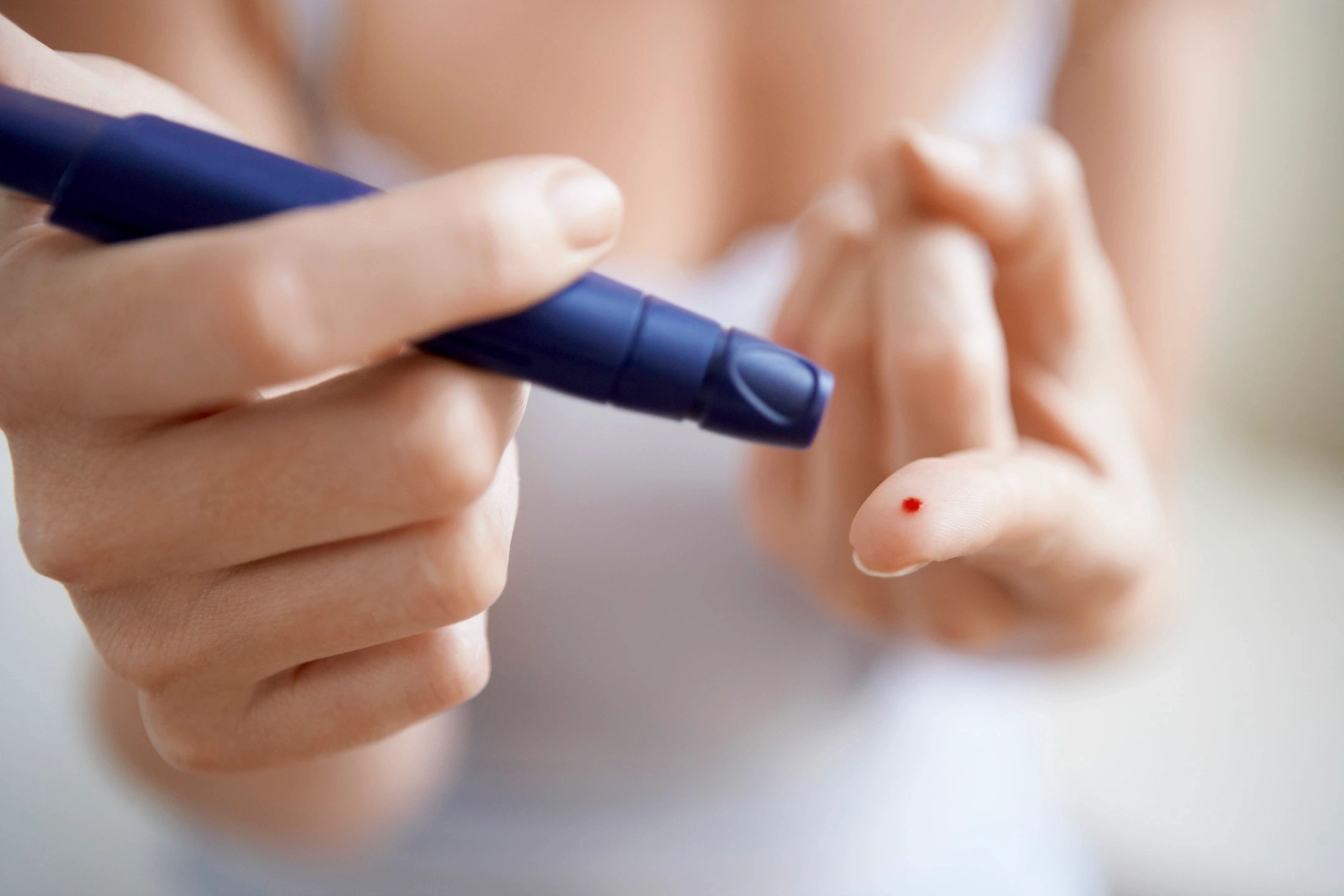
Project 6
C2CD5 role in energy balance and glucose homeostasis
C2CD5 is a protein characterized by the presence of a C2 domain, which is typically involved in calcium-dependent phospholipid binding. This protein has been found to play a crucial role in insulin-stimulated glucose transport, particularly in adipocytes and muscle cells, which are key tissues in the regulation of glucose homeostasis.
Role in Insulin Signaling
- Insulin Receptor Activation:
- Insulin Receptor (IR) Interaction: C2CD5 interacts with the insulin receptor, facilitating its activation and the downstream signaling cascade essential for glucose uptake.
- Phosphorylation Events: Upon insulin binding, C2CD5 is involved in the phosphorylation events that activate the insulin signaling pathway, including the activation of insulin receptor substrate (IRS) proteins.
- Akt Pathway:
- PI3K-Akt Signaling: C2CD5 is involved in the phosphatidylinositol 3-kinase (PI3K) and Akt signaling pathway. Akt activation is crucial for the translocation of glucose transporter 4 (GLUT4) to the cell membrane, which allows glucose uptake into cells.
Glucose Transport
- GLUT4 Translocation:
- Membrane Fusion: C2CD5 plays a role in the docking and fusion of GLUT4-containing vesicles with the plasma membrane, a critical step for insulin-stimulated glucose uptake.
- Vesicle Trafficking: It is involved in the intracellular trafficking of GLUT4 vesicles, ensuring their proper movement towards the cell surface in response to insulin.
- Adipocyte and Muscle Function:
- Adipocytes: In adipose tissue, C2CD5 helps mediate insulin-induced glucose uptake, contributing to lipid storage and energy balance.
- Skeletal Muscle: In muscle cells, efficient glucose uptake mediated by C2CD5 is essential for muscle function and overall glucose homeostasis.
Energy Balance
- Fat Storage and Utilization:
- Lipid Metabolism: By promoting glucose uptake in adipocytes, C2CD5 contributes to the conversion of glucose to triglycerides for storage. This process is crucial for maintaining energy reserves.
- Energy Expenditure: Proper glucose uptake in muscle cells, facilitated by C2CD5, ensures that glucose is available for ATP production during muscle contraction and physical activity, influencing overall energy expenditure.
- Insulin Sensitivity:
- Metabolic Health: C2CD5 activity is associated with improved insulin sensitivity, reducing the risk of insulin resistance, which is a hallmark of metabolic disorders such as type 2 diabetes and obesity.
- Inflammation and Stress Response: C2CD5 may also play a role in cellular responses to metabolic stress and inflammation, further impacting energy balance and glucose metabolism.
Implications for Metabolic Disorders
- Type 2 Diabetes:
- Insulin Resistance: Impaired function or expression of C2CD5 can lead to reduced insulin-stimulated glucose uptake, contributing to insulin resistance and the development of type 2 diabetes.
- Therapeutic Target: Enhancing C2CD5 function or expression could be a potential therapeutic strategy to improve insulin sensitivity and glucose homeostasis in diabetic patients.
- Obesity:
- Energy Storage: Dysregulation of C2CD5 can affect the balance between energy storage and expenditure, potentially leading to obesity.
- Weight Management: Strategies to modulate C2CD5 activity could help in managing body weight by improving glucose uptake and energy utilization.
Conclusion
C2CD5 plays a critical role in regulating energy balance and glucose homeostasis by facilitating insulin signaling and glucose transport. Its involvement in GLUT4 translocation and membrane fusion in adipocytes and muscle cells is essential for efficient glucose uptake, impacting lipid metabolism, insulin sensitivity, and overall metabolic health. Understanding the mechanisms by which C2CD5 operates offers potential therapeutic avenues for addressing metabolic disorders such as type 2 diabetes and obesity.
Meet the team
Virginie Mansuy-Aubert
Principal investigator
Chaitanya Gavini
Senior Post-doctoral fellow
Gwenaël Labouèbe
Senior scientist
Nadia Elshareif
PhD student
Laetitia Raux
PhD student
Nadine Eliasson
PhD student
Alison Gandelin
Master Student
Lisa Cuozzo
Master student
Sebastian Buitrago Hernandez
Master MD
In the MALab, we believe that ...
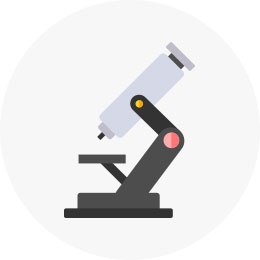
Science
is real
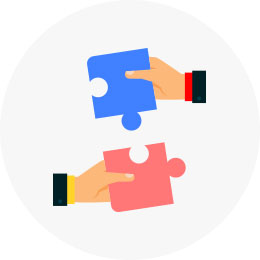
Teamwork
makes the dream work
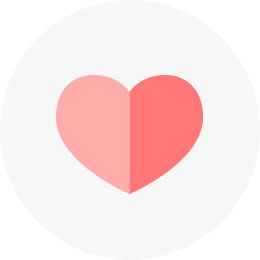
Love
is life
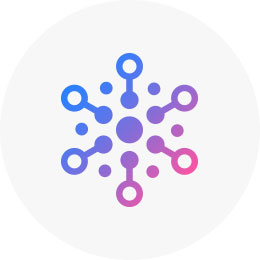
Sensory neurons
are cool
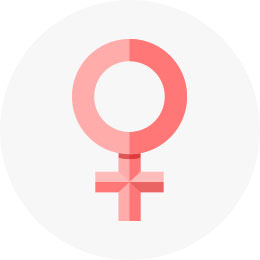
Feminism
is for everyone
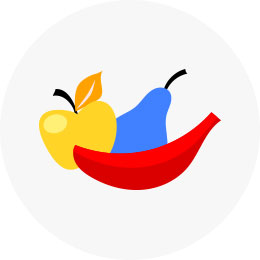
Feeding your microbiota
is all
Life @ the MAlab
Alumni
Valentin Barquisseau, senior scientist
Tyler Cook, PhD student 2017-2022, currently post-doc at Colorado University Anshutz
Raiza Bonomo, PhD student 2016-2020, post-doc at the NIH then FDA
Hollie Schaffer, Year 1, STAR medical student
Robin Wang, Loyola summer research program (2 months), Cornell College
Ryan Parnell, Case Western, Pharmadiscovery student
Shaima Patangia, MS Neurosciences, September 2018 – February 2018
Isabelle Brown, Year 3 Loyola U. Chicago, summer then one day per week
Trisha Cabrera, Year 2 Loyola U. Chicago, summer then one day per week
Sergio Escobar, Northeastern University, Summer PharmaDiscovery student
Shaima Patangia, Jan 2019 – June 2019
Robin Wang, Loyola summer research STAR program (2 months), Cornell College
Neha Jihian, SPARK high school program (2 months)
Nabihaa Khan, SPARK high school program (2 months)
Emilie Verran, Pre-med at Augustana college
Alisa Wang, SPARK high school program
Allan Sherman, SPARK high school program
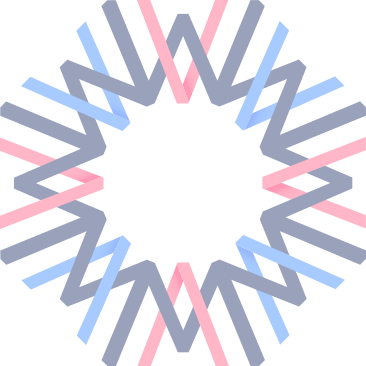